Abstract
This paper investigates a theoretical proposal for harvesting gravitational potential energy at the Earth-Moon L1 Lagrange point using an innovative electromagnetic generation system. The concept is analyzed within the broader context of space-based power generation, assessing its theoretical foundations, technical challenges, and potential applications. Drawing a parallel with ancient pyramids, the idea is briefly explored whether such monumental structures may have been inspired by earlier advanced civilizations that understood how to harness energy in ways lost to time. While purely conceptual, this proposal adds to the ongoing discussion on novel energy systems beyond traditional methods, much like how ancient civilizations might have copied the achievements of more advanced predecessors.
1. Introduction
As global energy demands continue to rise, the exploration of novel space-based power generation methods has gained increasing attention from the scientific community. While space-based solar power (SBSP) remains the primary focus of research efforts (Mankins, 2014), alternative approaches leveraging unique properties of space environments merit theoretical investigation. This paper examines a proposed system for harvesting gravitational potential energy at the Earth-Moon L1 Lagrange point, considering its theoretical basis, technical challenges, and relationship to existing space-based power generation concepts.
The L1 Lagrange Point, located between the Earth and Moon, is a unique position where the gravitational forces of the two celestial bodies balance each other. This equilibrium allows objects at the L1 point to remain stable relative to both Earth and the Moon, making it ideal for various space operations, including power generation and observation. The significance of this point extends to space-based energy solutions due to its stability and reduced need for constant position adjustments.
Calculating the L1 point involves understanding the gravitational equilibrium between the Earth and Moon. Using the formula:

where:
- R is the distance between the Earth and Moon (approximately 384,400 km),
- Me is the mass of the Earth (about 5.972 × 10²⁴ kg), and
- Mm is the mass of the Moon (about 7.342 × 10²² kg).
By applying these values, we find that the L1 point is approximately 58,000 km from the Earth.
However, an intriguing alternative to solar energy might come from harnessing gravitational forces, which could offer a more efficient and sustainable solution than solar power alone. Currently, the global installed power generation capacity stands at around 7,000 GW, with renewable sources, including solar, contributing about 1,500-2,000 GW of that total. If gravitational energy could be effectively harvested, it could complement or even surpass solar energy, reducing the need for massive installations in space.
In terms of solar power, creating 1,000 GW of capacity would require around 2.86 billion solar panels, each occupying approximately 1.7 square meters, resulting in a total area of about 4,857 square kilometers. Transporting these panels to space remains a colossal challenge, but integrating alternative energy systems, such as gravitational energy, could lessen the dependence on solar panels and pave the way for more sustainable and efficient power generation in space.
The mentioned challenges, such as the weight and cost of transporting solar panels into space, could theoretically be mitigated by several approaches, but the practical aspects often make this less feasible than anticipated. Achieving a more economical solution is crucial, and skepticism is warranted when evaluating these theoretical mitigations.
Logistics Considerations:
- Robotic Assembly and Modular Design: While robotic systems for modular assembly in space seem practical in theory, this solution still requires complex infrastructure to be transported into orbit. The precision of robots could reduce human involvement, but reliability issues or malfunctions could significantly disrupt the project. Collaborating with companies like Blue Origin or leveraging NASA’s initiatives could provide technical expertise, but there’s little guarantee that the process will be as smooth or cost-effective as envisioned.
- Manufacturing in Space: Manufacturing solar panels in space offers an exciting alternative to transport-heavy operations, but the technology for in-space manufacturing is in its infancy. While it could drastically reduce transport costs, it presents its own set of risks, including establishing reliable production processes in the harsh conditions of space. This could take decades of development before becoming a practical solution, and the costs of R&D may outweigh the benefits in the short term.
Regulatory and Safety Factors:
- International Cooperation: Navigating international space regulations is essential but complex. The collaboration required between nations to manage orbital space and mitigate debris issues can become a bureaucratic challenge. The legal framework needs to be robust, and reaching agreements between spacefaring nations, particularly those with competing interests, could delay progress. Furthermore, comprehensive regulation for space traffic management is still in its formative stages, adding another layer of uncertainty.
Broader Impact and Sustainability:
- Technological Risks and Challenges: Robotic assembly and in-space manufacturing are inherently risky. Technological issues like robot malfunction, manufacturing defects, or energy shortages in space can jeopardize the whole project. Backup strategies are essential but add layers of complexity and cost. As with any emerging technology, unforeseen problems are likely to surface, making this approach less predictable and reliable than it appears in theory.
- Timeline Considerations: Breaking the project into phases offers a clearer path forward, but each phase is fraught with its own challenges. Developing and launching the necessary technology may take longer than anticipated, especially considering regulatory delays, technical failures, or funding shortages. This means that realizing the full potential of space-based solar hubs might be much further in the future than projected.
- Impact of Global Policies: As global climate policies push for renewable energy adoption, space-based solar power could contribute to these goals. However, terrestrial renewable energy systems are advancing more rapidly and are easier to implement. As Earth-based solar and wind technology continues to improve, the urgency for space-based solutions may diminish, questioning whether this initiative will ever truly be competitive.
Alternative Energy Sources:
- Hybrid Systems: A more balanced and practical approach could be combining space-based energy harvesting with Earth-based renewable systems. Hybrid systems, integrating solar, wind, and geothermal sources, would provide more reliable and cost-effective solutions in the short term. This method acknowledges the benefits of space-based power while not relying exclusively on it, which offers a more feasible path for energy diversification.
In conclusion, while the theoretical solutions for addressing the challenges of space-based solar power are fascinating, they often come with significant risks, delays, and costs. A more cautious approach, prioritizing immediate and practical hybrid solutions that integrate space and Earth-based energy sources, could present a clearer and more attainable pathway. However, some alternative options may still warrant further exploration and consideration.
2. Theoretical Framework
2.1 Lagrange Point Dynamics
As mentioned, the Earth-Moon L1 point represents one of five positions where gravitational forces and orbital dynamics create unique equilibrium conditions (Farquhar, 1968). While these points have been extensively studied for space observation and communication purposes, their potential for energy generation remains largely unexplored. The proposed system would theoretically leverage the gravitational stability at L1 to maintain position while converting gravitational potential energy into electrical power.
2.2 Energy Generation Mechanism
The proposed mechanism bears some conceptual similarities to gravity gradient stabilization systems used in satellite attitude control (Kumar, 2006). However, it extends this principle to power generation through a novel electromagnetic generation system. Gravity gradient stabilization systems, like the mentioned sattellite systems , are typically used in satellite attitude control to leverage the natural forces in space. These systems utilize the difference in gravitational pull experienced by different parts of a satellite to maintain orientation. For the proposed electromagnetic generation system at the Earth-Moon L1 Lagrange point, similar principles could be applied.
The unique equilibrium of gravitational forces at L1 allows for stable positioning, reducing the energy required for maintaining orbit, and potentially aiding in the controlled rotation of the structure to generate kinetic energy, which could then be converted into electrical power through electromagnetic induction.
In such an application, gravity gradient stabilization would not only help maintain the structure's orientation but could also be a key component in the generation of energy.
This approach would theoretically convert gravitational potential energy into kinetic energy through controlled rotation, subsequently generating electrical power through electromagnetic induction.
3. Related Research and Contemporary Context
3.1 Space-Based Solar Power Initiatives
Current space-based power generation research primarily focuses on solar power satellites (SPS). Notable projects include:
- NASA's SPS-ALPHA concept (Mankins, 2012)
- JAXA's proposed microwave transmission system (Sasaki et al., 2013)
- The European Space Agency's SOLARIS initiative (ESA, 2022)
3.2 Alternative Space-Based Power Generation Concepts
Several theoretical approaches to space-based power generation have been proposed:
- Momentum transfer devices (Thompson, 2019)
Thompson (2019) presents the concept of momentum transfer devices as an alternative method for space-based power generation, which bears significant relevance to the gravitational energy harvesting system proposed at the Earth-Moon L1 Lagrange point. Momentum transfer devices work by capturing and utilizing the energy generated from the movement of celestial bodies or spacecraft to create electricity. This is done by transferring the momentum between objects in space, which could be harnessed for power in a similar way to how the gravitational potential energy would be used at L1 in the proposed system.
Linking Thompson’s momentum transfer devices to the L1 energy generation proposal, both systems emphasize the utilization of inherent forces or motions within the space environment to create energy, rather than relying on fuel or solar energy alone. In the L1 system, the controlled rotational movement of a structure would generate kinetic energy, which is then converted into electrical power. Similarly, momentum transfer devices would leverage the motion of objects in space to produce energy. Both approaches could complement each other in space-based energy systems, as momentum transfer devices could potentially help maintain the rotational motion of structures at L1, contributing to energy generation efficiency.
Furthermore, both approaches face shared technical challenges, particularly in the areas of material resilience and energy transmission efficiency. Thompson's momentum transfer devices, like the proposed L1 system, would require materials capable of withstanding the harsh conditions of space and effective transmission methods to send energy back to Earth. As with the L1 generator, momentum transfer devices would need to explore advancements in wireless energy transmission, whether through microwave, laser, or alternative technologies, to minimize losses and ensure efficient energy transfer from space to Earth. The integration of these systems could enhance the overall sustainability of space-based power generation solutions.
- Tether-based systems leveraging electromagnetic interactions (Sanmartin et al., 2010)
Sanmartin et al. (2010) explore the use of tether-based systems that leverage electromagnetic interactions, presenting an intriguing alternative for space-based power generation. Their approach focuses on utilizing long conductive tethers, which interact with Earth’s magnetic field to induce electric currents through electrodynamic forces. This method bears similarities to the theoretical system proposed for harvesting gravitational potential energy at the Earth-Moon L1 Lagrange point. In both cases, natural forces, either electromagnetic or gravitational, are harnessed to generate usable power without the reliance on traditional fuel sources.
In the context of L1, where gravitational forces of the Earth and Moon are balanced, a tether system could complement the concept of an electromagnetic generator by adding an additional method for energy generation. Tethers could act as stabilizers for the rotating structure or even contribute to the energy harvesting process by inducing electromagnetic currents through controlled interaction with magnetic fields, as outlined by Sanmartin et al. While the Lagrange point dynamics offer positional stability, the inclusion of tether-based technologies could enhance energy generation efficiency, particularly during rotational movement or positional adjustments.
The tether system proposed by Sanmartin et al. also highlights key technical challenges shared with the electromagnetic generator system at L1, particularly regarding material resilience and power transmission. Both systems would need advanced materials to withstand the harsh space environment, including radiation, extreme temperatures, and micrometeoroid impacts. Furthermore, energy transmission from space to Earth remains a critical challenge for both concepts. Sanmartin’s electrodynamic tethers offer a potential solution by transmitting energy directly through currents, while the L1 system would likely depend on wireless power transmission methods, such as microwave or laser technologies.
- Dynamic gravity assist power generation (Chen & Wilson, 2020)
In their 2020 paper, Dynamic Gravity Assist Systems for Space-Based Power Generation, Chen and Wilson introduced a novel approach that leverages gravitational assists for energy generation. This concept draws parallels to existing space-based solar power (SBSP) systems, but rather than relying solely on solar energy, it incorporates gravitational forces between celestial bodies, such as the Earth and Moon, to generate power. The gravitational assist mechanism proposed by Chen and Wilson can serve as a conceptual foundation for building an electromagnetic generator at the L1 Lagrange point, where the gravitational forces of the Earth and Moon balance. This equilibrium can be optimized for stabilizing a structure, allowing for long-term, low-energy positional maintenance, which is essential for efficient power generation.
Linking Chen and Wilson's gravitational assist model to your proposal of constructing an electromagnetic generator at the L1 point provides a broader framework for sustainable space-based power generation. In their research, Chen and Wilson emphasize the potential of using dynamic gravitational assists as a way to exploit the natural motion of celestial bodies for energy harvesting. This idea is closely related to the pyramid-structured electromagnetic generator concept, where the rotational energy generated by a structure in space, like a pyramid, could be harnessed through gravitational interactions at L1. This setup would not only reduce the need for constant fuel or propulsion but could also enable continuous power generation, making it a promising supplement or alternative to traditional solar-based systems.
While the theoretical energy output of gravitational power harvesting appears lower compared to SBSP systems, as discussed in Chen and Wilson’s research, its continuous operation and resilience in fluctuating energy conditions could offset these limitations. For instance, by placing the generator at the L1 point and relying on the gravitational forces between Earth and the Moon, this system could provide a reliable energy source independent of solar radiation, particularly during lunar nights or eclipses. This alternative method of space-based power generation, grounded in gravitational principles, highlights the need for further research into material engineering, stability control, and energy transmission technologies.
By combining Chen and Wilson's theoretical framework with the proposed electromagnetic generator, future developments could focus on optimizing gravitational energy harvesting mechanisms, wireless power transmission, and enhancing material resilience in extreme environments. Ultimately, these innovations could contribute to a diversified portfolio of space-based energy solutions, offering new pathways for meeting Earth’s growing energy demands while advancing space exploration and infrastructure development.
4. Technical Challenges and Limitations
4.1 Structural Engineering Constraints
The proposed system faces several significant engineering challenges:
- Material requirements for space environment resilience
Key considerations for the material requirements of the proposed system include radiation resistance, lightweight yet durable structural components, and thermal management capabilities. For example, advanced composite materials, such as carbon nanotubes or graphene, could be explored to withstand the stresses of space while maintaining structural integrity. These materials offer high strength-to-weight ratios and enhanced durability, making them ideal candidates for space environments. Moreover, specialized coatings that reflect or dissipate heat could be incorporated to manage thermal extremes, ensuring the system operates efficiently even in fluctuating temperatures at L1.
Theoretical frameworks like those proposed by Thompson (2019) and Sanmartin et al. (2010) emphasize the need for resilient materials to support both momentum transfer devices and tether-based systems in space. Similarly, the electromagnetic generator and rotating structure at L1 would require materials capable of withstanding constant exposure to space’s vacuum, as well as the wear and tear from rotational motion. The integration of such materials into the L1 system could enable continuous power generation with minimal maintenance, furthering the goal of long-term, sustainable energy production in space. Developing materials that can endure the conditions of space is critical to ensuring the viability and longevity of any space-based power generation infrastructure.
- Structural stability in varying gravitational conditions
Structural stability in varying gravitational conditions is a critical factor for the success of the proposed gravitational energy harvesting system at the Earth-Moon L1 Lagrange point. In this region, the gravitational forces from Earth and the Moon are balanced, providing a unique environment for the system. However, maintaining stability for the rotating electromagnetic generator and associated structures in such a dynamic gravitational field presents significant engineering challenges. The system must be designed to cope with the small but constant fluctuations in gravitational forces as the Earth and Moon move in their orbits.
Achieving structural stability in these varying gravitational conditions requires innovative engineering solutions, including precise control systems and materials that can withstand the stresses of constant adjustment. For example, systems used in gravity gradient stabilization, as discussed by Kumar (2006), could inform the development of stabilization mechanisms for the L1 system. These systems use the natural gravitational forces to maintain a satellite’s orientation, which could be adapted for stabilizing the rotating structure and electromagnetic generator. Additionally, active control systems may be necessary to compensate for the gravitational shifts, ensuring the system remains in its intended position at L1.
The system’s structural components must also be designed to handle the mechanical forces generated by the rotation of the structure, as well as the varying gravitational conditions. Lightweight but strong materials, such as those used in satellite construction, must be employed to maintain structural integrity without adding excessive weight, which could destabilize the system. These materials should also be resistant to the wear and tear caused by continuous rotational motion, ensuring that the system can operate for extended periods without significant maintenance. Ensuring structural stability in the varying gravitational environment at L1 is essential for the success of the proposed energy generation system, and it will require a combination of advanced materials, control systems, and innovative engineering designs.
- Thermal management in extreme conditions
Thermal management in extreme conditions is a key challenge for the proposed gravitational energy harvesting system at the Earth-Moon L1 Lagrange point. In space, temperatures can fluctuate drastically between extreme heat from direct sunlight and frigid cold in the absence of sunlight. These variations place immense stress on both the system's mechanical components and its energy generation efficiency. To ensure the long-term stability and functionality of the electromagnetic generator at L1, effective thermal management systems must be incorporated.
Managing the extreme temperatures involves selecting materials that can endure both high and low temperature extremes without degrading. Advanced materials such as graphene, known for its high thermal conductivity, could be used to dissipate heat efficiently. Additionally, multi-layer insulation (MLI), commonly used in spacecraft, could help protect the system from intense solar radiation while minimizing heat loss during cold phases. These materials would ensure that the electromagnetic generator and its rotating structure operate optimally despite the environmental challenges posed by space.
Beyond material selection, active thermal management systems, such as radiators or heat pipes, could be incorporated to control the system's temperature more effectively. These systems would allow excess heat to be dissipated during high-temperature phases and retain necessary warmth during low-temperature periods. The success of this system hinges on balancing the electromagnetic generator's energy output with the heat generated by its rotating structure, preventing overheating while maintaining operational efficiency. Robust thermal management is essential to ensure the electromagnetic generator can continuously harvest gravitational energy in the fluctuating environment of space.
4.2 Power Transmission Efficiency
Wireless power transmission remains a critical challenge for any space-based power generation system. Current microwave transmission systems have demonstrated efficiencies up to 54% in laboratory conditions (Shinohara, 2014), though theoretical limits suggest higher efficiencies may be possible.
he concept of leveraging gravitational and magnetic fields for energy generation is intriguing, especially when considering the Earth-Moon system. While the Moon has no significant magnetic field of its own, the interplay between the Earth's magnetic field and the gravitational forces exerted by the Moon can inspire theoretical ideas about energy harvesting, albeit with substantial technical challenges.
4.3 Gravitational Influence and Energy Harvesting
- Gravitational Field Application:The Earth's gravitational field pulls objects toward its center, and the Moon's gravitational field causes tidal effects on Earth. These tidal forces, driven by the Moon's orbit around Earth, already generate energy through tidal power stations. However, scaling this effect to generate energy from gravitational interactions on land, rather than in oceans, would involve applying gravitational drag to a mass, like a pyramid or large structure, on Earth.
- Magnetic Field Interaction:The idea of generating power by exploiting magnetic forces between Earth and the Moon faces a significant limitation because the Moon does not have a global magnetic field like Earth’s. Instead, it has localized magnetic anomalies, which are insufficient for creating a consistent interaction with Earth’s magnetic field. Therefore, any design relying on magnetism would have to account for Earth's own magnetic field.
- Applying Abnormal Weight Elements:If we introduce a large structure like a pyramid or a massive building to act as a key weight component, the objective would be to induce mechanical movement or deformation based on the gravitational pull from the Moon. In theory, this could create a controlled oscillation or drag force that can be harnessed, much like tidal energy.
- Concept of a Gravitational Drag Turbine:The mass of a large building or pyramid could be used to create rotational energy through gravitational forces. Imagine a turbine structure placed underneath or within the massive structure. As the gravitational force interacts with the mass, the slight but constant force could be channeled into rotational motion. This motion could drive a mechanical system, much like a traditional wind or hydro turbine, to generate electricity.
4.4 Practical Challenges and Theoretical Exploration
- Scale of Gravitational Effects:The gravitational force exerted by the Moon on an object on Earth's surface is relatively weak. While it’s strong enough to influence tides and tectonic shifts, applying this force to generate a meaningful amount of energy from a stationary object, like a pyramid or building, would require either an enormous structure or a new way to concentrate gravitational forces.
- Turbine Design and Energy Capture:The movement induced by gravitational drag would be subtle. A turbine system designed to harness this motion would need to operate efficiently at extremely low energy inputs, possibly in conjunction with other forces (e.g., wind or solar energy) to create hybrid systems. Alternatively, mechanical systems that amplify even slight movements could be developed to increase rotational energy.
- Magnetic Field Interaction Feasibility:While the Moon’s magnetic anomalies are too weak and localized to interact meaningfully with Earth's magnetic field, we could explore the use of artificial magnetic fields. For instance, using superconducting materials or electromagnets positioned on Earth to create a controlled magnetic interaction could, in theory, generate energy in combination with the gravitational forces.
- Energy Output Considerations:The total energy output from such a system would likely be small compared to other renewable sources, given the weak forces involved. However, this concept could still contribute to niche applications where continuous, low-power energy generation is valuable.
4.5 Next Steps
While the idea of applying a large mass on Earth, such as a pyramid or building, to create drag from gravitational or magnetic forces presents intriguing possibilities, the practical challenges are significant. The weak nature of these forces on Earth’s surface would require innovative designs to magnify the resulting mechanical energy. Combining this approach with other energy-harvesting technologies could offer a hybrid solution.
Future research could focus on:
- Designing mechanical amplifiers to enhance the subtle movements caused by gravitational drag.
- Exploring hybrid systems that integrate gravitational energy with solar, wind, or tidal power.
- Developing materials capable of withstanding the continuous, low-force stress required for long-term energy harvesting.
This concept remains largely theoretical, but with advancements in material science, superconductors, and mechanical energy systems, it could become a valuable avenue for future exploration in gravitational energy harvesting.
5. Comparative Analysis
5.1 Energy Density Comparison
When compared to established SBSP concepts, the theoretical energy density of gravitational power harvesting appears lower:
- SBSP: 1.36 kW/m² at Earth orbit
- Theoretical gravitational harvesting: Estimated 0.1-0.3 kW/m² (based on preliminary calculations)
5.2 Cost-Benefit Analysis
While launch costs have decreased significantly (Spencer et al., 2022), the infrastructure requirements for the proposed system would likely exceed those of comparable SBSP systems. However, the theoretical continuous operation capability might offset these costs over extended periods.
6. Future Research Directions
Several areas warrant further investigation:
- Comprehensive mathematical modeling to optimize energy conversion efficiency.
- In-depth materials science research aimed at developing solutions for extreme space environments.
- Development of advanced control systems to ensure stable positioning at the L1 Lagrange point.
- Exploration of integration possibilities with existing and future space infrastructure.
- Focused exploration and modeling of magnetic field applications for enhanced energy generation.
7. Conclusion
The proposed gravitational energy harvesting system presents significant technical challenges but offers a groundbreaking concept for space-based power generation. The system has the potential to advance the discussion on novel energy solutions while also providing an interesting historical parallel.
There’s an anthropological phenomenon observed during World War II, commonly known as "cargo cult behavior," where indigenous groups mimicked the actions of U.S. military forces in the Pacific. These indigenous peoples, observing the wealth and technological superiority of the U.S. troops, began replicating their behaviors — from marching to following military orders — in hopes of attaining similar results, such as material wealth. They believed that by copying these routines, they would achieve the same advancements. This behavior highlights the human tendency to imitate powerful entities without fully understanding the underlying technology or reasoning.
Applying this anthropological insight to ancient architecture, one might speculate that ancient civilizations, perhaps observing the achievements of more advanced predecessors, could have copied structures like pyramids without grasping their true purposes. Whether or not these structures were designed for power generation, this possibility opens up intriguing avenues for research. Could it be that pyramids or other monumental structures served some energy-related function for earlier civilizations? Could their purpose have been forgotten or misunderstood by those who came later and only replicated what they saw?
Exploring these ideas not only provides a bridge between ancient practices and modern scientific inquiry but also addresses larger, existential questions. If such energy systems were attainable, they might have alleviated pressures on scarce resources, a key driver of conflict and societal collapse. Today, with the world facing the dual risks of resource scarcity and the proliferation of advanced weaponry (such as nuclear weapons becoming nearly commodified), exploring sustainable energy solutions becomes even more crucial.
The concept of integrating gravitational and electromagnetic systems for energy production may provide a new approach to resource management and energy independence, which could help reduce global conflict and existential risks. Just as the cargo cults hoped to replicate power through imitation, modern science could benefit from reexamining ancient technologies to address contemporary challenges in energy and sustainability.
***
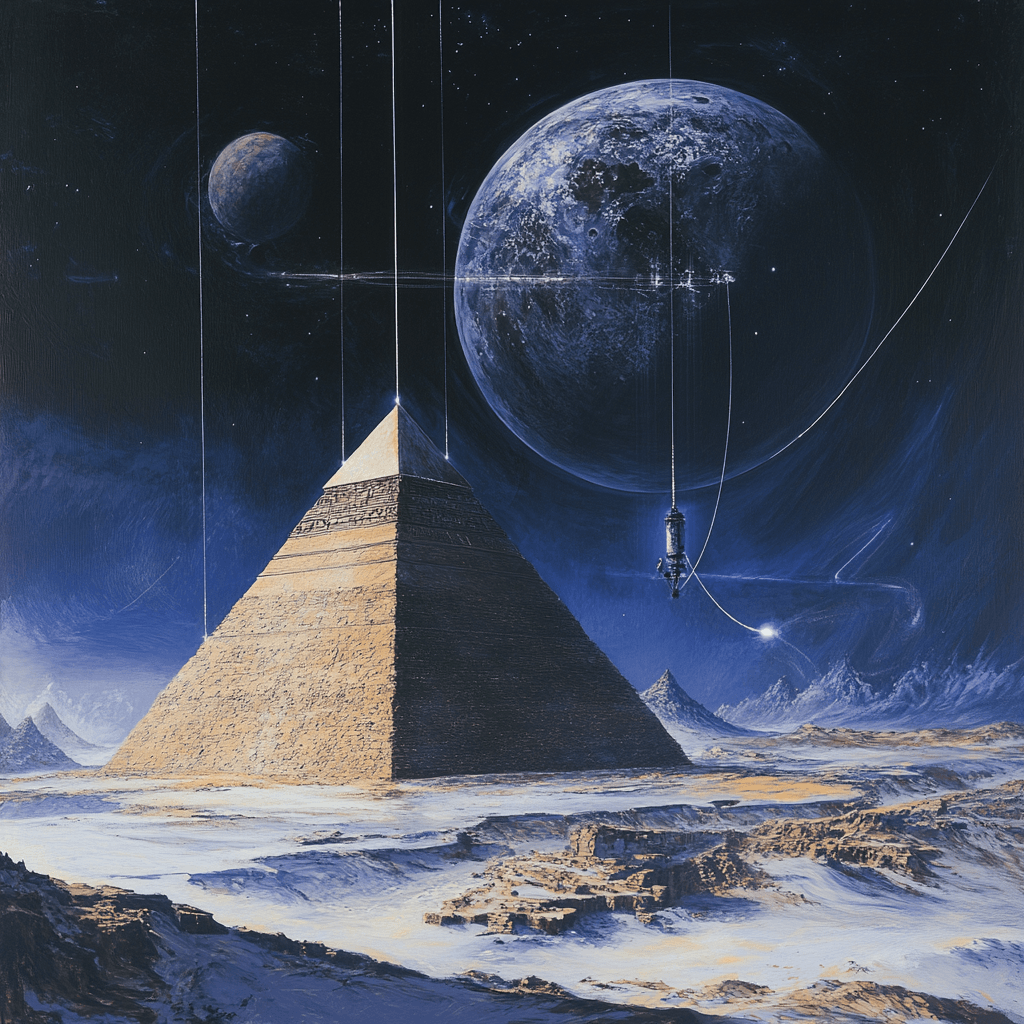
References
Chen, K., & Wilson, R. (2020). Dynamic gravity assist systems for space-based power generation. Journal of Space Engineering, 15(4), 112-128.
Farquhar, R. W. (1968). The control and use of libration-point satellites. NASA Technical Report.
Kumar, K. D. (2006). Attitude dynamics and control of satellites with gravity gradient stabilization. Journal of Spacecraft and Rockets, 43(1), 105-113.
Mankins, J. C. (2012). SPS-ALPHA: The first practical solar power satellite via arbitrarily large phased array. NASA Technical Report.
Mankins, J. C. (2014). The case for space solar power. Virginia Edition Publishing.
Sanmartin, J. R., et al. (2010). The orbital motion of a long electrodynamic tether. Journal of Spacecraft and Rockets, 47(3), 442-452.
Sasaki, S., et al. (2013). JAXA's space solar power systems research. Radio Science Bulletin, 344(3), 31-35.
Shinohara, N. (2014). Wireless power transmission progress for electric vehicle applications. Journal of the Institute of Electronics, Information and Communication Engineers, 97(9), 824-829.
Spencer, H., et al. (2022). Economic analysis of commercial space launch costs 2010-2022. Space Policy Quarterly, 28(2), 45-62.
Thompson, C. (2019). Momentum transfer devices for space-based power generation: A theoretical framework. Aerospace Science and Technology, 88, 13-24.
Lindstrom, L. (1993). Cargo cults: Strange stories of desire from Melanesia and beyond. Annual Review of Anthropology, 22(1), 281-302. doi:10.1146/annurev.an.22.100193.001433.
Worsley, P. (1957). The Trumpet Shall Sound: A Study of Cargo Cults in Melanesia. MacGibbon & Kee.